SMITHSONIAN, March 1984, Volume 14, Number 12, pages 56-67
Engineers versus the eons, or How long will our monuments last?
By James R. Chiles
Illustrations by Paul M. Breeden
Even if we abandon them, giant structures might endure as long as the Seven Wonders; one may even make it to the next Ice Age
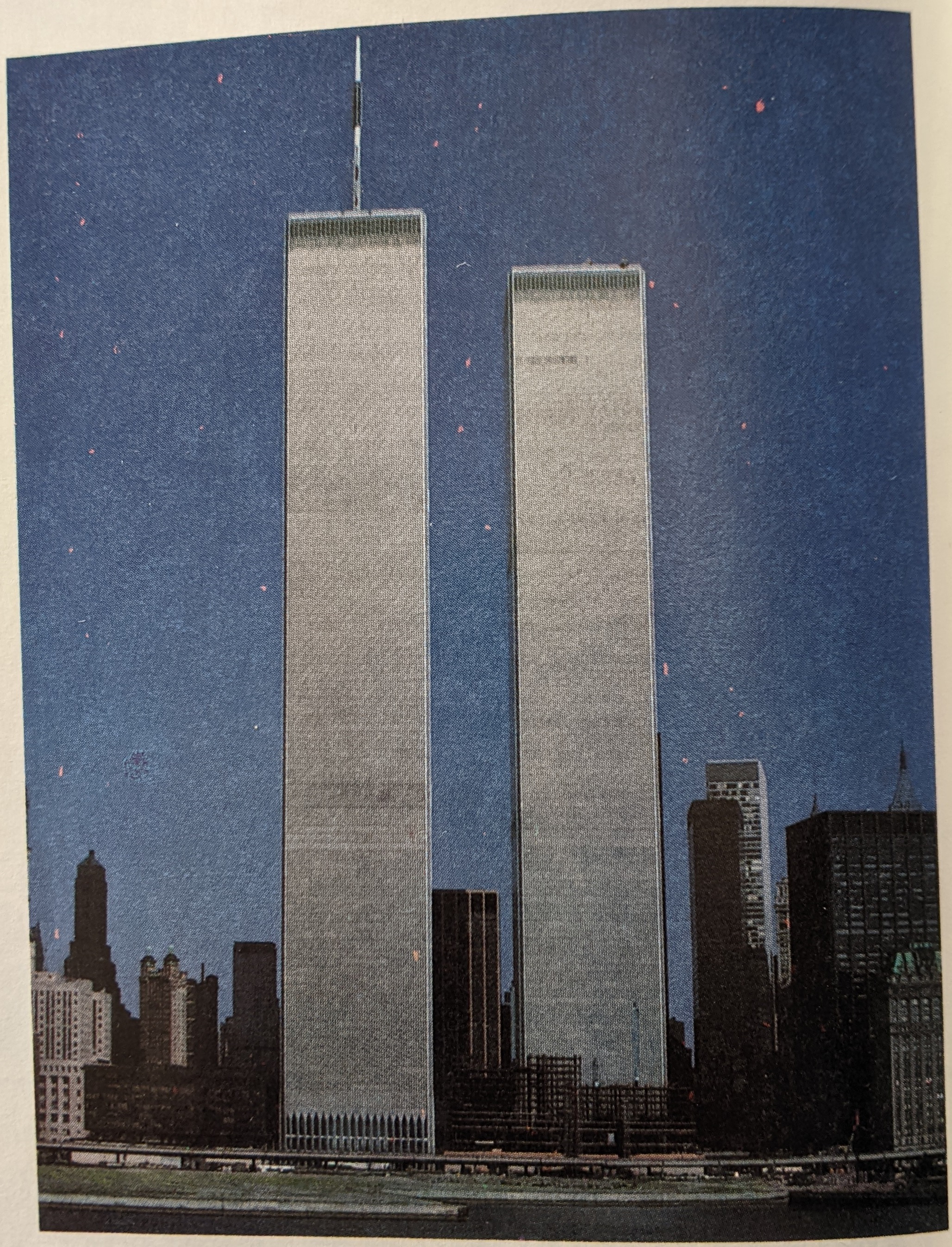
If all goes according to plan, one day in 6939 a crowd will gather in New York City’s Flushing Meadow. Workers—probably a squad of robots—will bore 50 feet into the soil. They will haul up a copper cylinder containing ten million words and 1,000 pictures on microfilm: parcel post to the future from the World’s Fair of 1939.
Americans didn’t invent the time capsule. Sumerians and Egyptians put inscriptions and mementos in the foundation deposits of important buildings. Something in human nature hopes we’re not just chalk marks on the blackboard of eternity.
One way we reach for influence beyond our life span is with our buildings, particularly our monumental structures. Most crumble in a geologic instant. Of the Seven Wonders of the World, six are no longer with us. At least two fell to earthquakes (one just 55 years after it was built), and one to war. We are not sure what happened to several others, and there is doubt that one ever existed. The best of the six lasted about 1,500 years. The seventh wonder, and also the oldest, is still with us: the 4,500-year-old pyramids at Giza.
Some modern wonders are already suffering. Acid rain is burning through the Statue of Liberty’s torch and copper gown. Weather, road salt and the pounding of trucks crumble the concrete decks of our bridges. Constantly we repair, we replace, we restore.
How would some of America’s grandest structures measure up if we all packed our bags and left? The question is not as far-fetched as it sounds: if any structure stands long enough, odds are good that the parent culture will abandon it to the erosion of earthquakes, fire, weather and war. The pyramids, Stonehenge, the Great Sphinx, Machu Picchu, Mesa Verde: all of these have been untended most of their lives. Their declines are measured in hundreds and even thousands of years.
My aim is to predict the independent life of three modern structures, entirely apart from human hands that can help or hurt. The structures: the twin towers of the World Trade Center of New York City (above), the tallest skyscrapers in the world save one; the Gateway Arch of St. Louis, the nation’s highest monument (p. 60); and the Grand Coulee Dam of Washington State, the largest concrete structure on Earth (p. 64).
I peered off roofs, pored over plans and poked at rust. I went to the structural engineers, maintenance men and geologists, and asked: “What if we abandoned this thing? What is the sequence of events?”
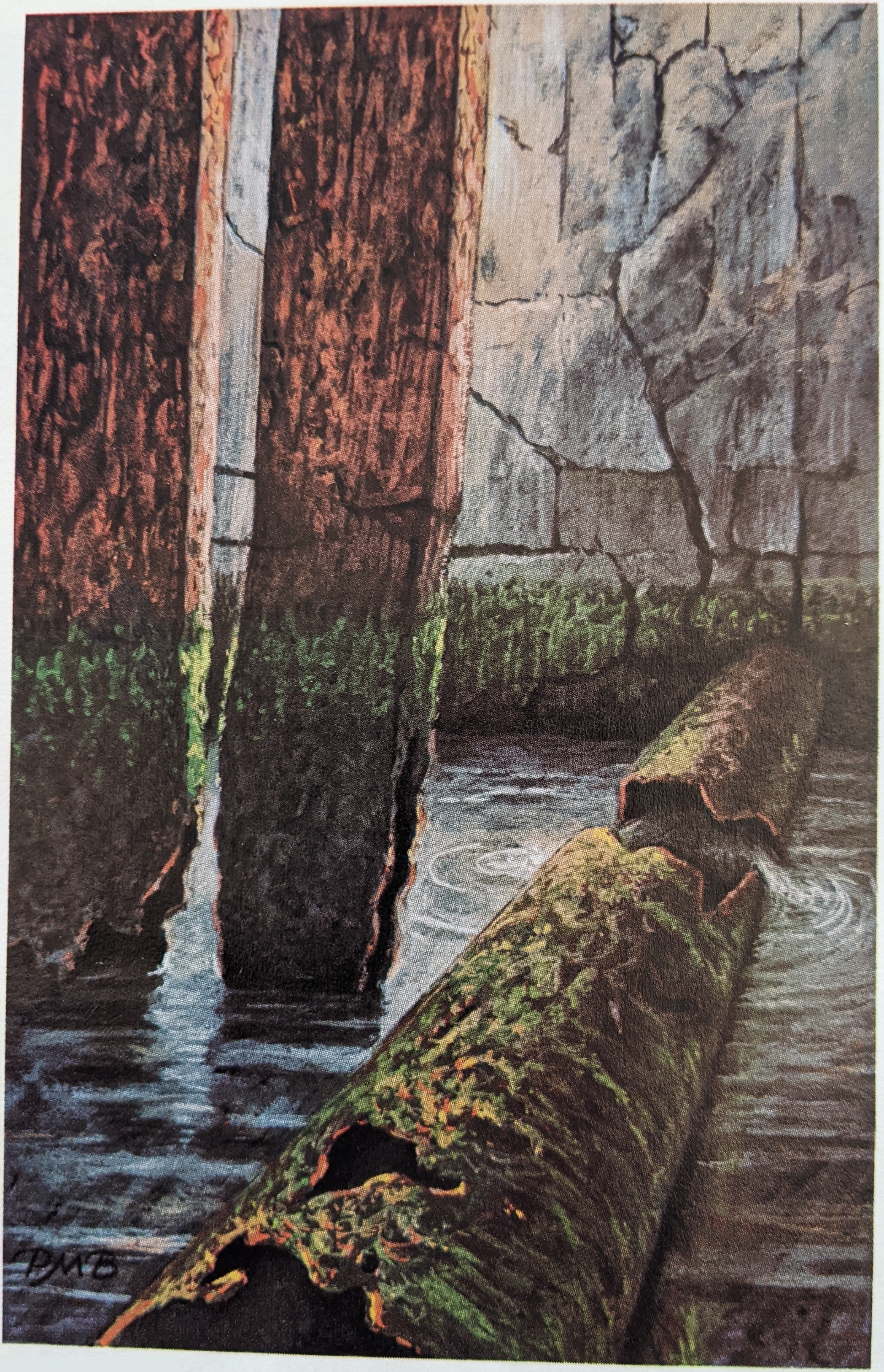
The World Trade Center towers are easy to describe (SMITHSONIAN, January 1978). They loom over the Hudson River, on the southern end of Manhattan Island. At the top, 1,350 feet and 110 stories up, the towers are the same width as at the base, still square plan, each floor nearly an acre in area.
Saving the towers from blockishness is a fine lattice of columns that reach from plaza to thick concrete roof. Tall, narrow windows peek out between them. From a distance the columns appear delicate, but they are the towers’ strength against winds. Wide welded-steel plates, called spandrel beams, connect the columns horizontally at every floor and help stiffen them. Aluminum covers protect columns and spandrel beams from weather.
Something has to hold the floors up. In the Trade Center towers, the external steel walls share the load with a separate set of steel columns in the core of each building. Together, the core columns and external wall columns pass the 280 thousand tons of each building 68 feet down through the basement to bedrock schist.
How might such a building die if everyone walked off? Les Robertson of New York had some speculations.
Robertson is the structural engineer who designed the steel-and-concrete frame of the World Trade Center towers. His firm used tables and charts, wind-tunnel tests and computers to put a skeleton into architect Minoru Yamasaki’s vision. Lean and silver-haired, seated in his conference room, Robertson cast his mind forward into the future and imagined how his greatest work might die.
Almost surely within a hundred years, perhaps as soon as 20 years, the basements would fill with salty water (left) from the Hudson River after heat-exchanger supply pipes or one of the PATH transit tubes rusted through. This is very important. As Robertson said, “Filling of the basements would be the biggest single danger to the Trade Center.” The reason is rust. But nothing major happens for several centuries, so we’ll return to the rust later.
What of the windows? Even the best-behaved buildings lose a few windows now and then. Winds stir up gravel from adjacent roofs and nick the glass, which eventually fractures and later breaks out. Once this process starts, it is self-reinforcing. Wind plays through the floors and breaks out more windows. Over centuries, gasketing would embrittle under the influence of sun and heat. High winds would pop the glass out.
Buildings last longer without windows
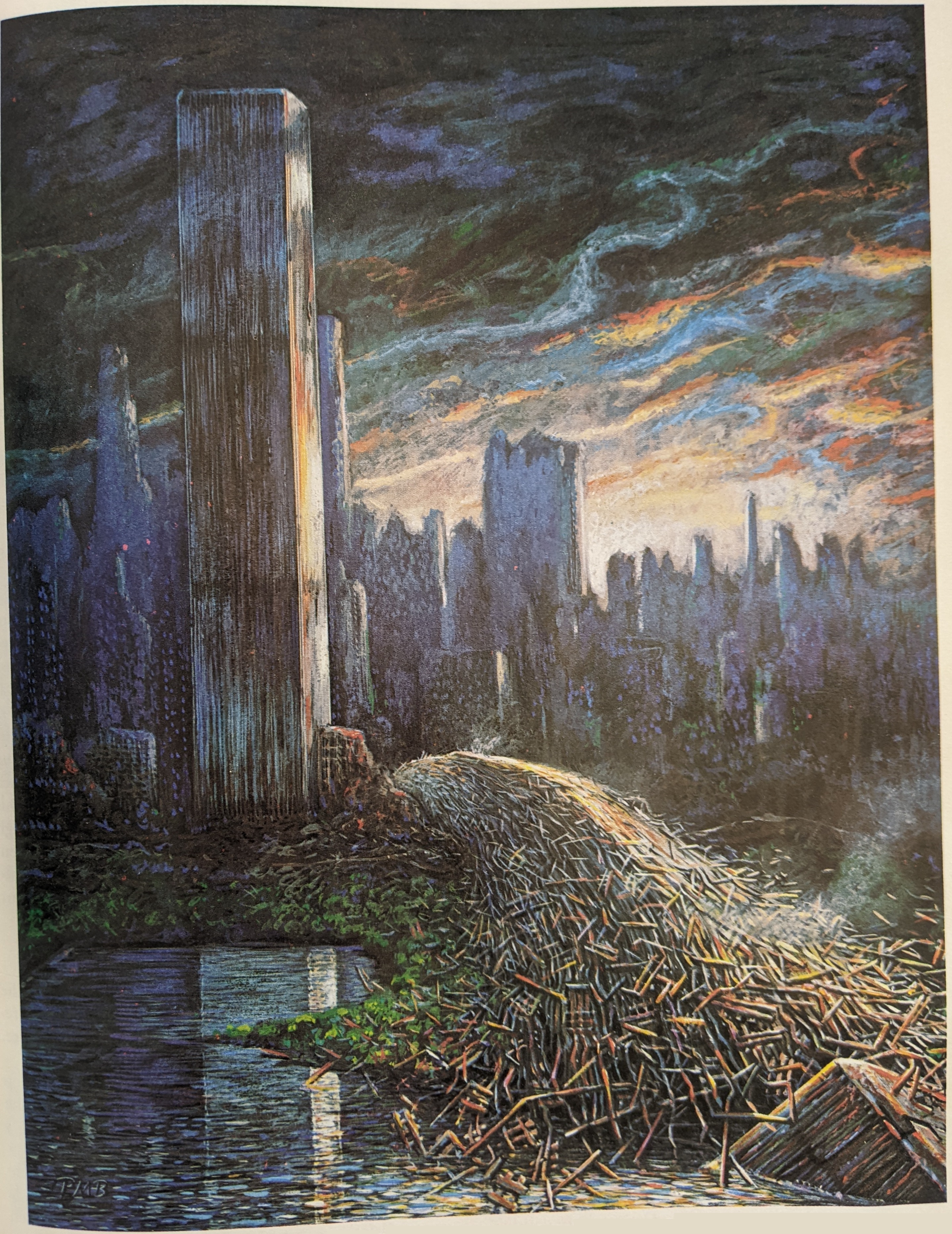
Oddly, said Robertson, “as the glass strips out of the buildings, the buildings themselves become more resistant to the wind,” the ultimate destructive force. Empty windows diminish the wind’s impact.
The loss of glass would help, but it could not keep the towers up forever if important supports in the basement were being eaten by rust. Rust is nature’s way of returning steel to iron ore by combining it with oxygen. Oxygen is richest at the tidal zone, where water rises and falls daily. The basement of the Trade Center would have a tidal zone, because the broken pipes or tubes would admit and discharge water from the tidal Hudson River.
In the basements are three types of structural steel: the core columns under the central areas of the buildings, the bases of the external wall columns, and diagonal braces. In cross-section all are hollow boxes of structural steel, with walls about three to eight inches thick.
In a strong enough wind, the rusted and separated structural steel in the basement might fail in one of several ways, causing the rest of the building to fall over. The diagonal bracing could collapse, or the external wall columns on the basement’s downwind side could buckle under the compression that high winds cause. Or, less likely, the upwind external wall could rip apart under tension. “Failure mechanisms are very complex,” Robertson cautioned. He said he has no firm idea how long failure might take, but doubted that the towers would stand much beyond a thousand years if abandoned. He thought a hurricane might finish off the abandoned towers.
Picture how the fall might happen: hurricane winds push at the gap-toothed building with the force of 15,000 tons or more. In the basement a few rusty columns bend; other columns take the load, but it’s too much to bear. More buckle and the building shudders like a notched tree. Moving very slowly, it leans a little more downwind; concrete explodes off other columns in the basement. Moss-covered furniture in the lobby slides as the ground floor dips. Pigeons and owls flutter out of broken windows into the hurricane and are swept away.
Skyscraper and tenants crash to the ground
Shortly after the tower begins to fall downwind, it also starts collapsing into the basement. A sound like gunfire rattles the air as bolts connecting the steel wall panels shear off. Most of the remaining windows shatter. Concrete floor panels rumble as they crack in a hundred places. A screeching builds in a crescendo, louder than the howling of the hurricane wind, as hundreds of steel columns and spandrel beams tear in half. The tower is picking up speed now, 500 feet off the vertical, leaving an arc of debris across the air. Cats, rats, cockroaches—all tenants go into free fall. Skyscraper greets ground with a mighty roar and the Trade Center tower is no more. Not much later, the second tower would fall. End of scenario.
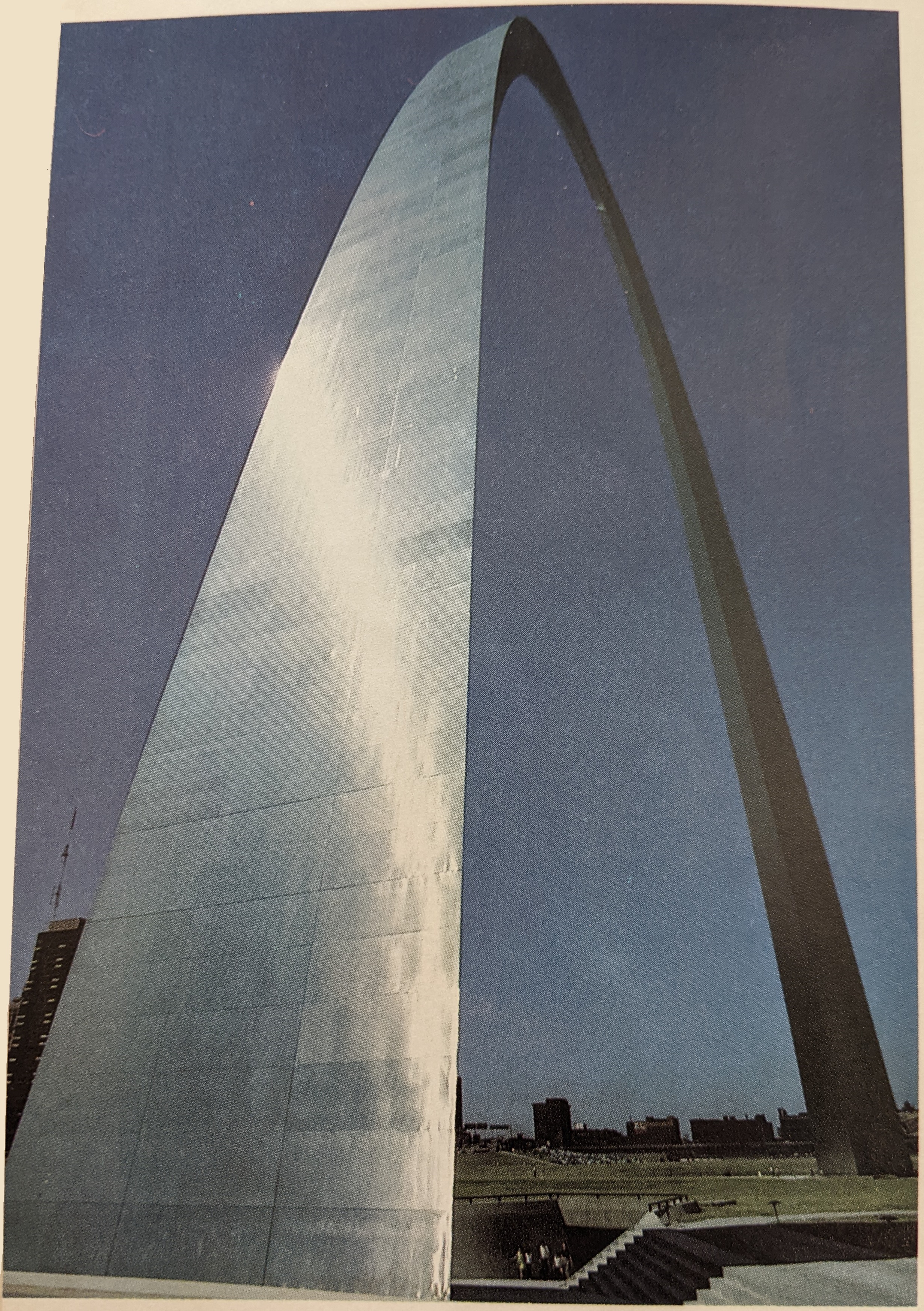
Wind would also be an accomplice in the death of the Gateway Arch, but the modus operandi is considerably different. The 18-year-old arch is a breathtakingly slender structure, soaring 630 shiny feet from a bluff located between St. Louis and the Mississippi River. Federal and city money built it to celebrate the city’s role in the settlement of the West.
In cross-section the Gateway Arch is an equilateral triangle with 54-foot sides at the base and 17-foot sides at the top. It has two skins spaced a short distance apart. The outer skin is butt-welded stainless-steel plates. The inner is carbon steel. Short steel rods span the space between the two skins. The arch is stiffened by a concrete filling between the skins from ground level up to the 300-foot mark.
Anchored in each base’s 13,000-ton concrete foundation are hundreds of long steel post-tensioning strands that reach up to grasp the concrete in the legs. Some strands extend to the top of the concrete.
“No metal used is free from corrosion”
The arch’s stainless-steel skin is steel with nickel and chromium added to the melt during its manufacture, which significantly increase its durability and hardness. In the presence of water, however, “no metal used in building structures is entirely free from corrosion,” said Geoffrey Frohnsdorff, chief of the Building Materials Division of the National Bureau of Standards. In fact, even new stainless steel is protected by a thin, transparent film of oxide similar to rust. But over long periods the busy fingers of water and abrasion by airborne dust rub this oxide off and more stainless steel reacts to take its place.
If the arch were abandoned to the weather, little would happen for many years. Tibor Szegezdy, who is managing partner of the firm that was the arch’s structural engineer, thinks that a structure of this size and significance should be periodically surveyed. Szegezdy said that eventually the corrosive combination of water and dissolved acids would etch small openings in the stainless-steel exterior. These would permit water to get under the arch’s skin (right).
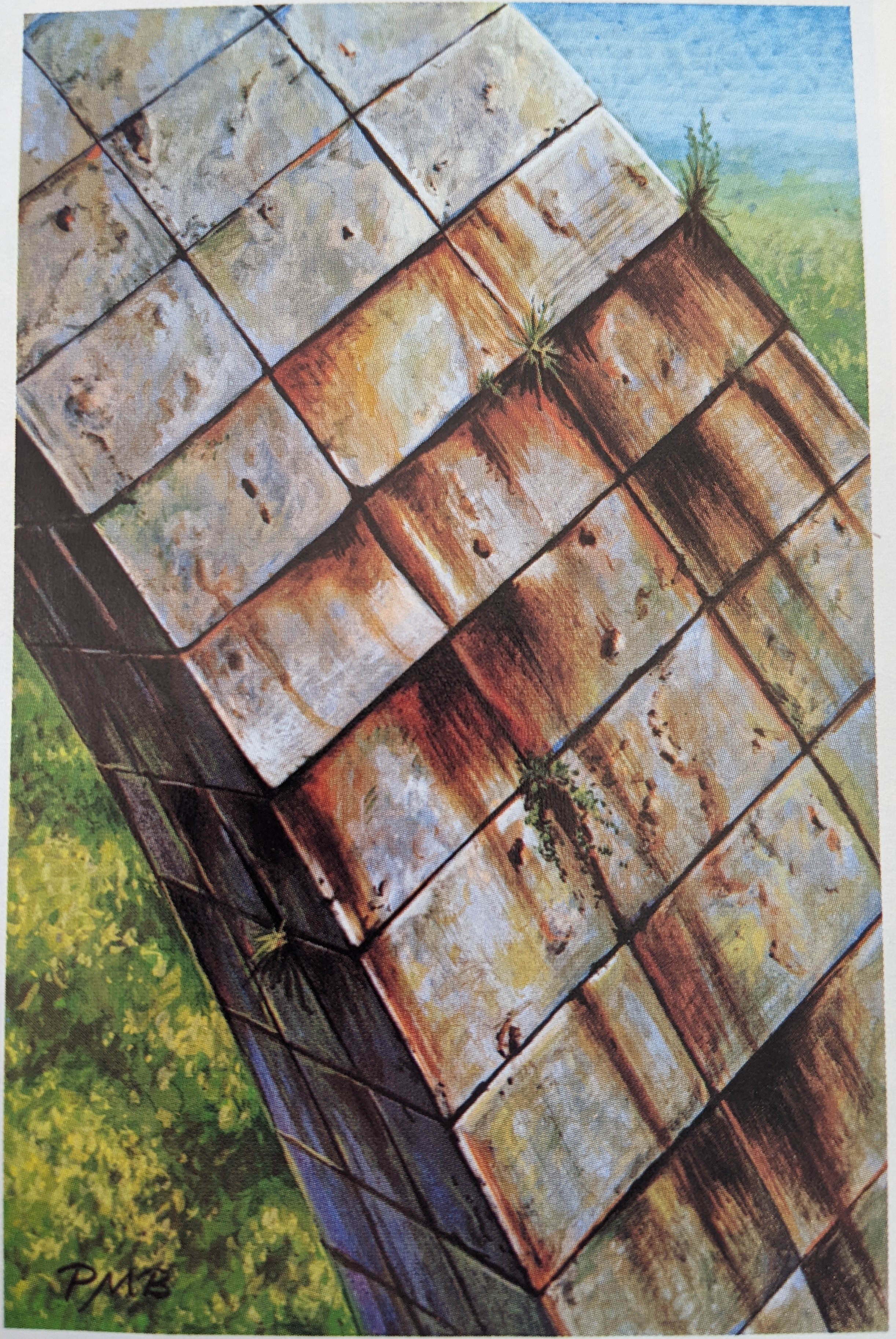
Water would run down to accumulate on and deteriorate the top of the concrete filling, 300 feet above the ground. It would also rust the steel stiffeners between the skins there, and the inner carbon-steel skin. Szegezdy thinks that a weakness would develop at the 300-foot level, as cracks appeared in the steel skins.
What would fell the arch? One of North America’s strongest earthquakes, called the New Madrid after one of the Missouri towns it shook down, occurred 172 years ago. The active zone reaches to 150 miles south of the arch. It spawns big quakes every 650 to 700 years. And earthquakes, said Szegezdy, would be similar to high winds in their effect on the arch. Would a quake do the job?
Not likely, Szegezdy said. “The wind loads are of much greater concern than earthquake loads here, because the area is known for high winds and the probability of an earthquake is relatively low. The arch, because it is tall and slender, is more susceptible to wind.” Indeed, a tornado passed within a mile of the present site of the arch in 1874. Szegezdy guessed the arch, if not maintained, would stand “considerably less than a thousand years.”
A scenario: one summer day hundreds of years from now, warm winds rise to wave branches on the trees growing on crumbled buildings of downtown St. Louis. The arch, shiny but spotted in places, stands temporarily triumphant over the leafy skeletons of masonry. To the southwest, greenish-black clouds gather. A funnel forms, touches the ground to take on a dirty tint and vacuums a sinuous path toward the arch. The top of the arch starts swaying in a nervous ellipse. The tornado passes 500 feet to the west and 120-mph winds shove on the steel sides. The top half of the arch leans a bit too far and folds over at those rusty cracks to collapse on the ground. As the tornado roars off for smaller prey, the two concrete-stiffened arms jut 300 feet into the air, as if reaching for their missing link (p. 62). The pillars might stand for another 5,000 years.
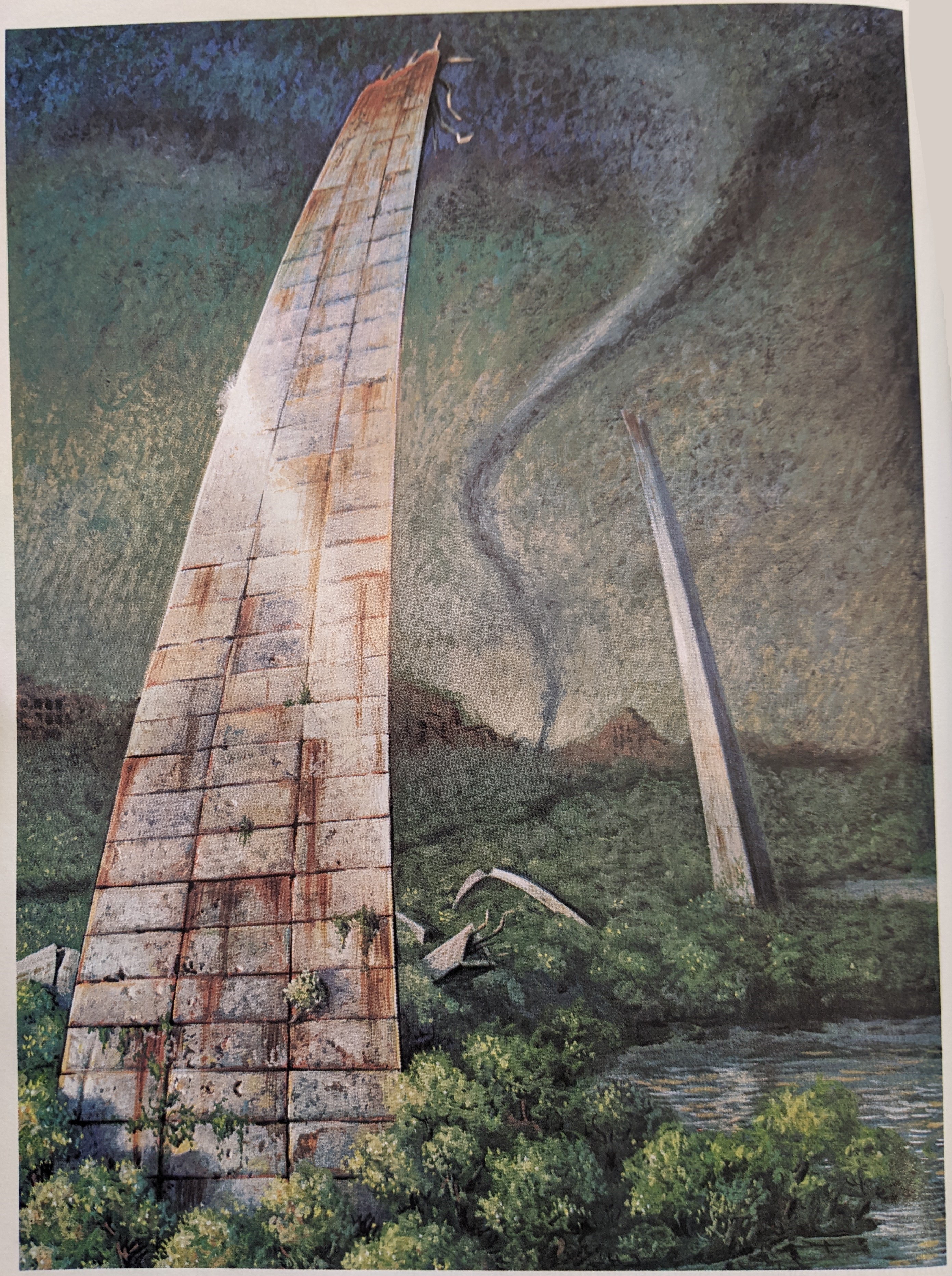
That’s a long time, but Grand Coulee Dam on the Columbia River would be around even longer. This monolith of eastern Washington State has the durability that only 24 million tons of concrete can give. Major parts would survive until the next Ice Age. Glaciers would destroy it, but not in the way you might expect.
Properly designed, dams are durable. A 20-foot-high stone dam on Syria’s Orontes River is more than 3,200 years old. By comparison, Grand Coulee is but a toddler; it first started lighting bulbs in 1941.
The dam once ran straight as a string across the valley. There’s been some work since then, and now it takes several doglegs. Starting from the west side, it emerges from the granite valley walls, runs about 4,000 feet straight across, turns downstream for about 1,000 feet (the “forebay dam”), then turns to meet the opposite side of the valley in a short section 200 feet long (the “wing dam”). The dam’s owner, the U.S. Bureau of Reclamation, added the last two sections in the 1970s to bring water to six big new generators at the base of the forebay dam.
The massive dimensions of a grand dam
At its tallest, the dam is 550 feet high; at its thickest, 500 feet through. It isn’t quite solid; it’s mazed with nearly ten miles of galleries, stairways and shafts.
Drawing water from the lake are 24 main turbine generators, three service generators for internal electrical housekeeping, and six pumps and six pump generators on the west end. The three largest turbine generators each harness a million horsepower with rotating parts weighing 3,000 tons apiece. They take their water through 40-foot diameter tubes called penstocks that tap the upstream base of the newest part of the dam.
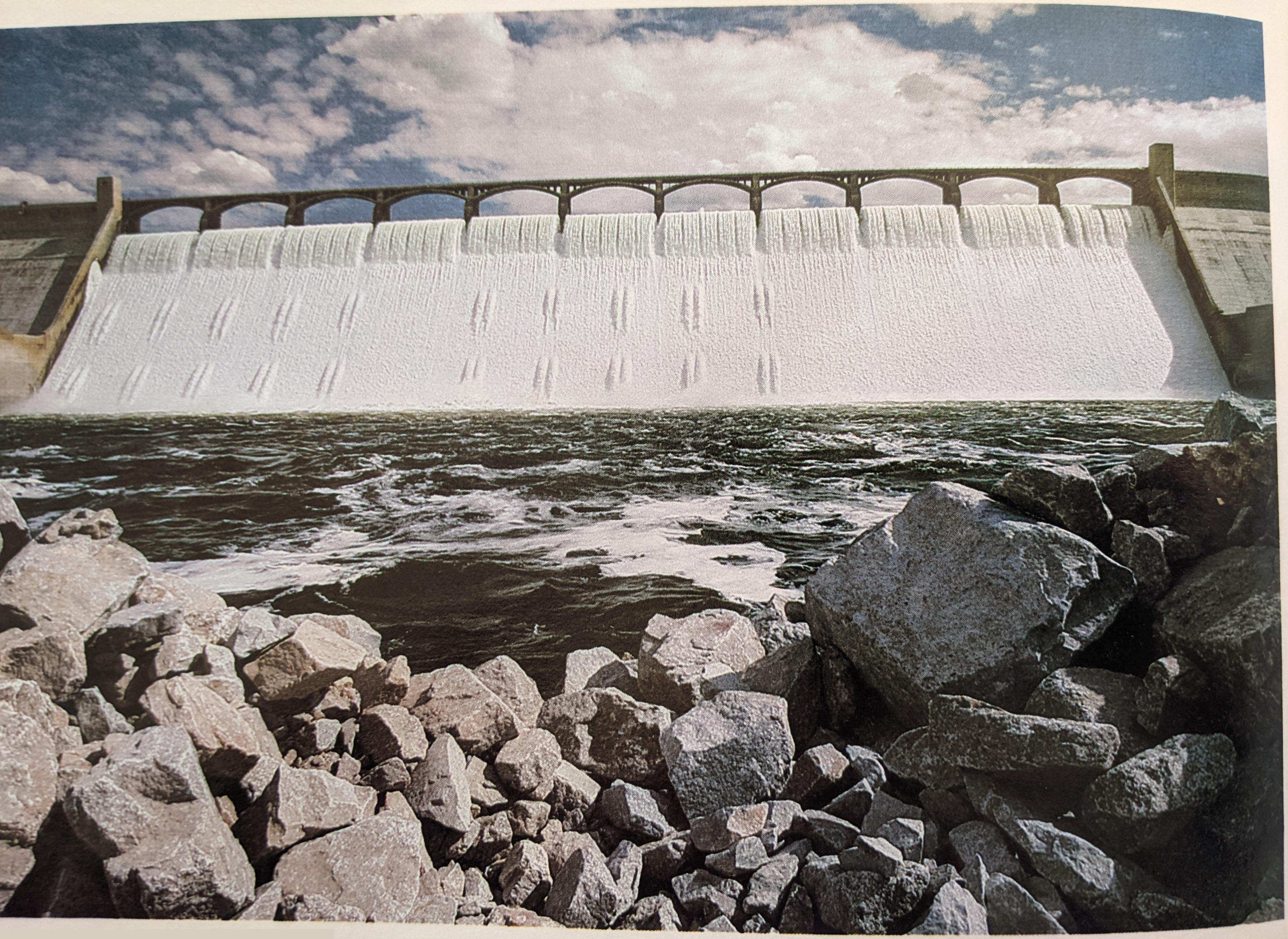
I posed The Question to some Grand Coulee engineers and geologists familiar with the area. “You mean you want to know what would happen if somebody blew a whistle and we all took our lunch boxes and went away for good?” asked Steve Sauer, chief of Grand Coulee’s mechanical engineering branch. Exactly, I said. I added the premise that consumers would stop using electricity. They agreed to speculate, with the caution that other experts might conjure a different sequence.
“If you took the load off the power grid,” said Jerry Pederson, chief of the water and power operations branch, “you’d start losing the main generators in days.” As the power requirements fell, the resistance against turning would drop and the generators would spin too fast. Automatic controls would shut gates that allow water to flow in from the lake.
These gates are very important to what eventually would happen to the turbine generators. The six newer generators can protect themselves without human intervention: they would lower gates where water first enters the penstocks. They would also use hydraulic pressure to close a second set of gates that surround their turbines. These are called wicket gates, and they rotate open and closed like vertical Venetian blinds. Operators use them to fine-tune the amount of water reaching the turbines.
The 18 smaller, older generators are not as clever. They can close the wicket gates around their turbines, but only human operators can close the penstock gates to these generators. As long as station service power held out, hydraulic pumps would stubbornly keep these penstock gates up. The result: water in the 18-foot diameter penstocks, just waiting for the wicket gates to open.
Probably within a week, then, all main generators would have rolled to a halt. Only the three station service generators would still turn. They would supply power to hydraulic pumps, air compressors, lubricating oil pumps, water pumps in the sump pits of the dam, emergency battery chargers and lights. “The station service generators could last for months,” said Pederson. “Among the three, at least one could go for months—a couple of years, even.”
Moments after the last station service generator shut down for lack of maintenance, relays would tap a huge bank of batteries to power emergency lights and hydraulic pumps. After a few hours the batteries would die; darkness and silence would fall over the powerhouse. For the last time?
Generators rumble to life in the dark
No. Remember the wicket gates on the 18 older generators? These gates need hydraulic pressure to stay closed. Without power “they’ll open back up to a 35 percent setting,” said Jerry Pederson. That’s not a lot, but the generators would be electrically isolated now. The turbines would be free to spin without opposition from the generators’ electromagnets. It would be a sight worthy of any science fiction movie: in the dark and dusty powerhouses, all those generators that weren’t shut down for maintenance at abandonment would rumble to life, as if answering some instinctive call to destruction.
Destruction, because the pumps that normally force oil between the thrust bearing and the rotating shaft would have no station service power; they cannot draw electricity from the main generators they service. Friction would heat the bearings quickly.
“A bearing failure is pretty scary,” Pederson said. “Your first thought is to run. It starts hammering. If you’re standing nearby, it’s like somebody is hitting you on the soles with an eight-pound double-jack.” In a bearing failure the heat-expanded tin coating of the bearing shaves off.
There’s more. As shavings from the bearings wedged the first turbine generator to an abrupt halt, many tons of water would still be tumbling down the penstock every second. Pederson speculated that the pressure and mechanical damage “might destroy the head cover on top of the turbine … in that case, water would come up through the breach, onto the floor, and pour out the windows and doors of the powerhouse … it’s a real possibility.”
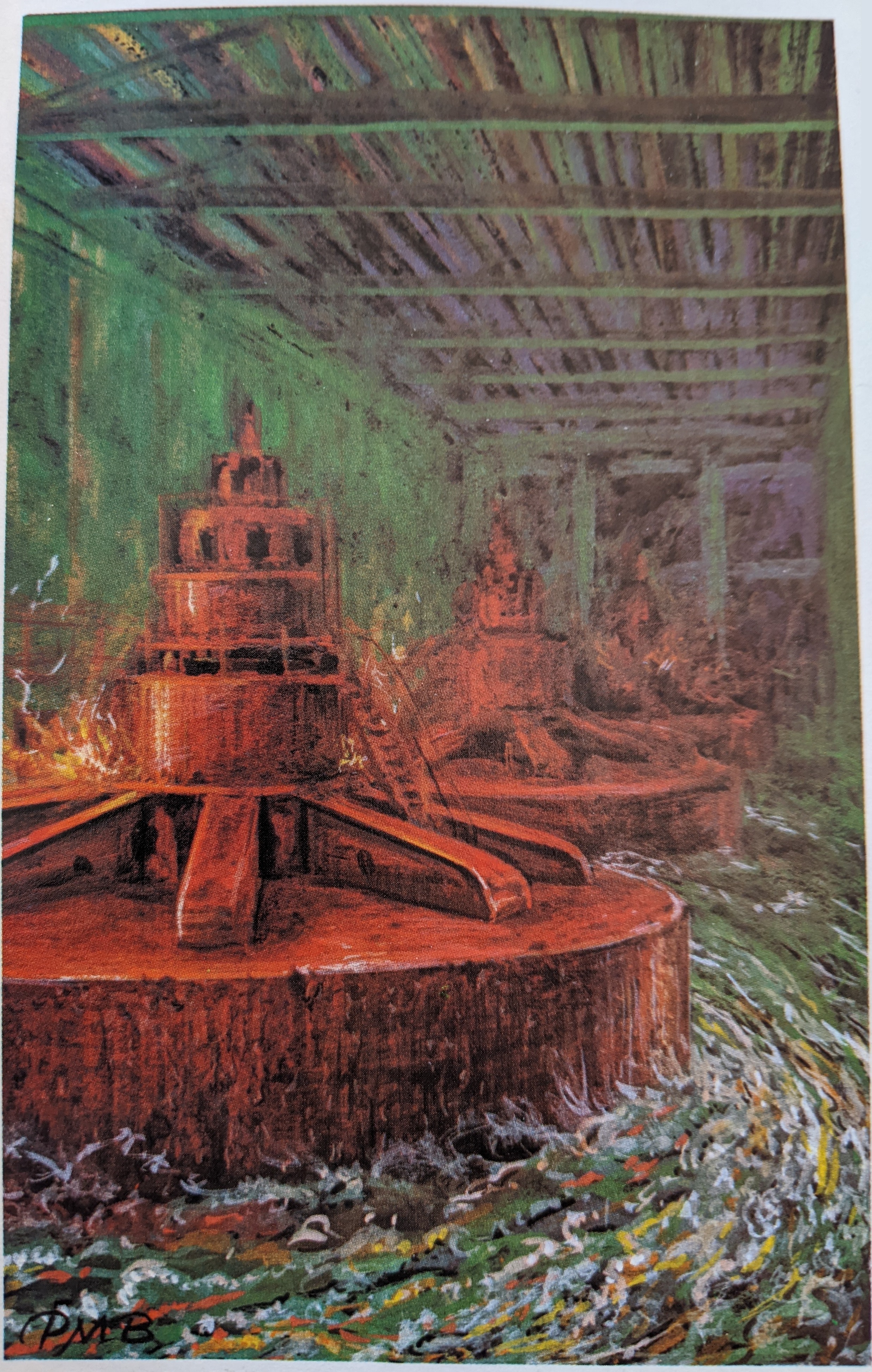
In that case, the escaping water would flood the turbine bearings of any units still running (p. 66). The water would float away any bearing oil that remained and help stop them.
Dams are not isolated structures. They rely on the integrity of dams upstream. Over several hundred years, chances are good that some extremely wet season would overtop one of the abandoned earthfill dams upstream. Overtopping is usually disastrous to earthfill dams; it starts gouges that keep getting bigger until all the water runs out.
Mica Dam, across the Canadian border and about 400 miles up the Columbia River, is one of the world's highest earthfill dams. “If a large geologic event should reduce that dam,” said civil engineer Bob Miller, “there's no reservoir that could contain all that water.” Phil Hansen, the dam's chief geologist, believed that Grand Coulee Dam would emerge still standing after such a flood. But he guessed that the sides of the dam, at the abutments, would show scouring. “The right [east] abutment is probably the most vulnerable part of the dam,” said Hansen. “Erosion there will make its own spillway.”
If after 10,000 years or so water eroded the entire wing dam away to expose the granite bench underneath, there would still be water in the lake because the bench is 240 feet higher than the riverbed. Water would spill over the eroded east end of the dam, and down the bench to the riverbed. But most of the dam would be standing.
What might happen to Grand Coulee Dam after this depends on the next Ice Age. The last one in the Northwest peaked 12,000 to 14,000 years ago, according to radiocarbon dates from the Seattle area. “The next Ice Age might finish it off,” said Brian Atwater of the U.S. Geological Survey, “not just from bulldozing by glacial ice, but also from great floods that came from an ice-dammed lake in Montana.”
Atwater referred to geologic evidence that during the last Ice Age a glacier blocked the Clark Fork River of western Montana to back up a tremendous lake: Lake Missoula, now vanished. At the peak of the last Ice Age, Lake Missoula held about 2,000 cubic kilometers of water, equal to 250 San Francisco Bays. Much of this huge volume of water occasionally escaped through Lake Missoula's glacial dam.
What did this water look like when it reached the present-day site of Grand Coulee? When modern dams break, like the St. Francis Dam near Los Angeles in 1928, there isn't enough water to sustain a high wave very far downstream. But the flood front from Lake Missoula was awesome. Geologist J Harlen Bretz wrote in 1969 that even 200 to 300 miles downstream from Grand Coulee, the flood front must have raced as a cresting wave at least 700 feet high. The legacy of such waves includes deep canyons cut into basalt—the largest of which is the Grand Coulee.
And even after this monster wave, the water kept coming, perhaps for weeks. How deep was the water at the site of Grand Coulee Dam? The floodwaters probably reached a 2,500-foot elevation, wrote geologist Bretz in 1932. That would be 1,600 feet deep over the current riverbed, and 1,200 feet over the crest of Grand Coulee Dam. And not just one flood but probably dozens because, according to Richard B. Waitt jr. of the Geological Survey, Lake Missoula issued at least 40 floods during the last Ice Age alone.
Explode the dam like a hand grenade
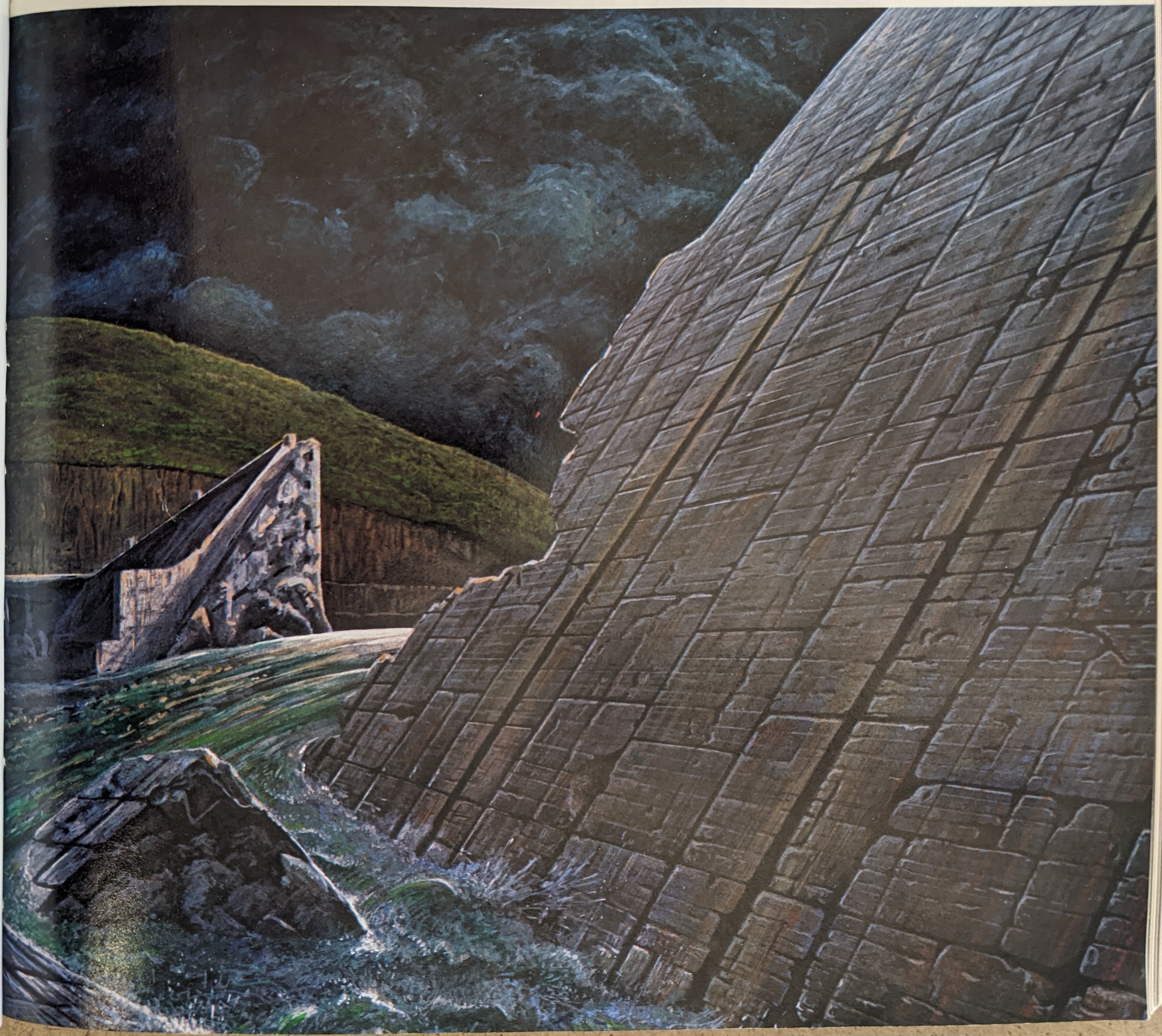
The impact of a wave 700 feet high might tear Grand Coulee Dam right off its foundations. Because of construction joints in the concrete, the dam would probably break into 50-foot-square columns like the grooved sections of a bursting grenade. Chunks of concrete might fly thousands of yards. And if anything were left of Grand Coulee Dam after the first dozen Missoula floods of the next Ice Age (opposite), Phil Hansen pointed out, then a glacier might come along and bulldoze out the remaining concrete.
If geology repeats itself, floods and glaciers will come to Grand Coulee again. How long before the next Ice Age? Maybe as few as 20,000 years, or as many as one million years. “It requires a substantial glaciation to cause Lake Missoula-style flooding,” said Larry Hanson of Eastern Washington University at Cheney. “Although there have been many floods during the more recent glaciations, there is less evidence for flooding prior to 100,000 years ago. In the long term, this kind of flooding may be a rare event.”
Most of the ancient Wonders of the World survived less than 1,000 years. The World Trade Center and the Gateway Arch, if abandoned, would probably last the same range of time. That is pretty impressive, considering their slenderness and height. The ancient Wonders were squat by comparison.
Our big concrete dams, like Grand Coulee, will give the pyramids tough competition for the title of longest-lived artificial structures. If you are placing a bet, though, better put your money on the pyramids. They do not sit in a riverbed. These artificial mountains might pass a million birthdays and outlast mankind.
Our country’s longest-lasting structure would probably be the deeply buried North American Aerospace Defense Command combat operations facility near Pikes Peak in Colorado. Protecting the steel buildings in their blasted-out caverns is a 1,700-foot-thick granite roof called Cheyenne Mountain.
Meanwhile, we’ll have to be content with buildings and bridges and dams that deteriorate rather quickly without upkeep. Structural engineer Les Robertson had something to say on this. “The idea of a 50- or 100-year life span for buildings is patently ridiculous,” he said. “There’s no structural reason not to go on and on and on.” He explained that any piece—and he means any piece—of a steel building can be pulled out and replaced. “The effective life span is completely dependent on those who maintain them. Properly maintained, a building is ageless.”
Six ancient wonders that did not survive | |
---|---|
![]() |
![]() |
![]() |
![]() |
![]() |
![]() |